Theories of Aging
One of the current ideas on aging is the free radical theory. Excess free radicals primarily from reactive oxygen species damage DNA, protein, and lipids. This ongoing free radical damage is called oxidative stress which is linked to mitochondrial dysfunction and ongoing inflammation. These three cellular mechanisms are involved not only in aging but are a common theme in chronic illness.
Additional ideas about aging involve the shortening of the telomerase enzyme leading to shortening of telomeres. Methylation is a common metabolic pathway for many reactions in the body. Changes in methylation, especially in DNA methylation influence aging. Genetic polymorphisms exist in methylation pathways which may explain different susceptibilities to diseases associated with aging. Finally, we are learning more about epigenetics and how the environment changes our DNA, often through methylation leading to aging.
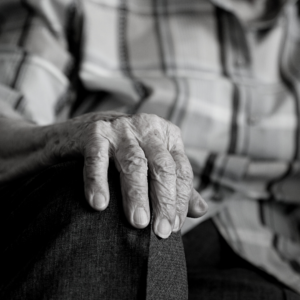
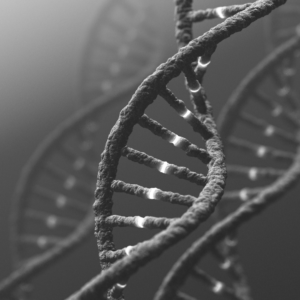
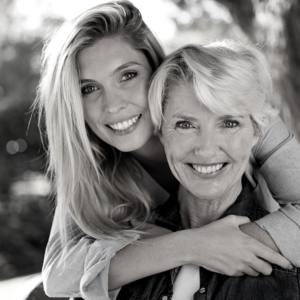
Mitochondria changes with aging
Mitochondria are an integral part of the free radical theory of aging. The production of energy requires the mitochondria to produce ATP. A normal part of this process is the formation of free electrons that react with oxygen to form the superoxide anion. The superoxide anion can then be converted into the more damaging free radicals; the hydroxyl radical and hydrogen peroxide.
As the mitochondria age, they undergo physical changes such as altered morphology and changes in quality with resultant decrease in energy formation. The mitochondria also have a conductance pore called the mitochondrial permeability transition pore (MPTP) which is closed in non-stressed conditions. With an increase in reactive oxygen species or an increase in calcium concentration the MPTP opens. Upon opening, the mitochondrial membrane loses its electrical potential causing the mitochondria to swell and rupture releasing pro-apoptotic factors that destroy the mitochondria.
With decreased effectiveness in the electron chain, more free oxygen radicals are released. The mitochondria are especially sensitive to the destructive effects of these free radicals leading to damaged mt DNA, proteins and lipid peroxidation. This creates a downward cycle of increased free radical production and decreased energy production called oxidative stress and mitochondrial dysfunction. The higher the energy demands of the organ, the higher the risk of mitochondrial dysfunction.
Cardiac mitochondrial changes
It is well known that cardiac disease increases with age and is a leading cause of disability and death. Cardiovascular disease ranging from ischemia/reperfusion injury, ventricular hypertrophy, myopathy, to congestive heart failure has been shown to involve dysfunctional cardiac mitochondria2.
Cardiac cells contain two types of mitochondria. The subsarcolemmal mitochondria are below the plasma membranes and are more involved in protein synthesis1,3. The interfibrillar mitochondria are located between the myofibrils. Their role involves more ATP production1,3. Three morphological changes are seen with aged cardiac mitochondria. The shape becomes rounder and more elongated leading to a decreased area of the inner membrane limiting the electron transport chain ability to form ATP2. A decrease in cardiolipin in the inner mitochondrial membrane contributes to this dysfunction. Acetyl-l-carnitine is a normal part of the mitochondrial membrane and helps restore cardiolipin3.
Skeletal mitochondrial changes
Sarcopenia or age related loss in skeletal muscle mass and function is another dreaded complication of aging. Even beginning as early as the fourth decade. Similarly, to cardiac muscle, skeletal muscle has two types of mitochondria: the subsarcolemmal mitochondria and the interfibrillar mitochondria. Oxidative damage from free radicals damage both types of mitochondria contributing to gradual loss of muscle function4.
There is truth to the saying if you don’t use it, you lose it. Even down to your mitochondria. Skeletal muscle decreases in amount and strength but some of this can be lessened by training. Part of what is lost in function is due to an increase in non-functional depolarized mitochondria and a decrease in mitochondrial density5.
Clinical studies show a correlation between a decrease in walking speed in the elderly with a decrease in mitochondria capacity6.
Adipose tissue mitochondrial changes
Adipose tissue functions as an endocrine organ producing adipokines such as adiponectin. These chemicals interact with cardiac and skeletal muscle. Mitochondria are needed for the secretion of adipokines7. Mitochondrial dysfunction in adipose tissue triggers systemic insulin resistance and cardiac dysfunction8. A decrease in fatty acid oxidation is seen with this dysfunction leading to an increase in cytosolic free fatty acids and damage to organs8. Obesity has been shown to accelerate aging. Mitochondrial dysfunction most likely plays a role through the adipose tissue.Brain mitochondrial changes
The brain has high energy needs. This translates into a large quantity of mitochondria with unique actions adapted to the nervous system. In healthy neurons, mitochondria supply ATP to the nerve terminals by moving from cell body to axons, dendrites, and then synapses.
Mitochondria then reverse their direction and travel back to the cell body from synapses9. Mitochondria have been found to be involved in many neurodegenerative diseases including Alzheimer’s disease, Parkinson’s disease, Huntington’s disease and ALS10,11. In Alzheimer’s disease part of the mitochondrial dysfunction is because of mitochondria that are unable to travel up and down the neurons12. Oxidative stress causes damage to mitochondrial DNA and enzymes leading to dysfunction and mitochondrial structural changes 9,10.
Anti-aging for your mitochondria
The formation of energy from the food we eat to the production of ATP requires many nutrients. Many of these nutrients can be supplemented in our diet as we age. CoQ10 is needed for the function of the electron transport. Many medicines, such as cholesterol lowering medicines lower COQ10 levels and contribute to mitochondrial dysfunction. Carnitine is another nutrient often lowered by prescription medicines. The B vitamins, especially Riboflavin and the NADH form of niacin are critical cofactors. The anti-oxidant lipoic acid works with the B vitamins in the breakdown of macronutrients13. Essential minerals such as magnesium are important. Many people are deficient in many of these important nutrients needed for mitochondrial function.
One other critical piece of supporting mitochondria is repair of the mitochondrial membranes. The formation of energy requires an intact double membrane in the mitochondria. Reactive oxygen species damage these membranes through lipid peroxidation. In order to repair these membranes, our body requires phospholipids including phosphatidylcholine. Research has shown improvements in mitochondrial function plus symptomatic fatigue using supplements containing targeted phospholipid replacement therapy14.
In today’s world, exposure to chemicals in our food and environment will contribute to oxidative stress proceeding to mitochondrial dysfunction. Everyday wear and tear will accelerate the effects of exposure. Therefore, avoiding aging is not possible. Understanding the biochemical pathways of mitochondrial energy production and oxidative stress gives us hope. It is possible to decrease damage by decreasing toxic exposures and increasing antioxidants. Supplementing our diets with healthy foods and targeted supplements along with exercise is a doable strategy to promote mitochondrial health and delay aging.
- Boengler K. Et al. Mitochondria and ageing: role in heart, skeletal muscle and adipose tissue. Jour of Cachexia, Sarcopenia, and Muscle. 2017 April.
- El’darov Ch M, Vays VB, Vangeli IM, Kolosova NG, Bakeeva LE. Morphometric examination of mitochondrial ultrastructure in aging cardiomyocytes. Biochemistry (Mosc) 2015;80:604–609.
- Paradies G, Petrosillo G, Gadaleta MN, Ruggiero FM. The effect of aging and acetyl-L-carnitine on the pyruvate transport and oxidation in rat heart mitochondria. FEBS Lett 1999;454:207–209.
- Short KR, Bigelow ML, Kahl J, Singh R, Coenen-Schimke J, Raghavakaimal S, et al. Decline in skeletal muscle mitochondrial function with aging in humans. Proc Natl Acad Sci U S A 2005;102:5618–5623.
- Lenk K, Schuler G, Adams V. Skeletal muscle wasting in cachexia and sarcopenia: molecular pathophysiology and impact of exercise training. J Cachexia Sarcopenia Muscle 2010;1:9–21.
- Coen PM, Jubrias SA, Distefano G, Amati F, Mackey DC, Glynn NW, et al. Skeletal muscle mitochondrial energetics are associated with maximal aerobic capacity and walking speed in older adults. J Gerontol A
Biol Sci Med Sci 2013;68:447–455. - Koh EH, Park JY, Park HS, Jeon MJ, Ryu JW, Kim M, et al. Essential role of mitochondrial function in adiponectin synthesis in adipocytes. Diabetes 2007;56:2973–2981.
- Medina-Gomez G. Mitochondria and endocrine function of adipose tissue. Best Pract Res Clinic Endocrinol Metab2012;26:791–804.
- Reddy PH. Et al. Mitochondria as a therapeutic target for gaining and neurodegenerative disease. Curr Alzheimer Res. 2011 June;8(4):393- 409.
- Lin MT, Beal MF. Mitochondrial dysfunction and oxidative stress in neurodegenerative diseases. Nature. 2006; 443:787–795. [PubMed: 17051205]
- Reddy PH. Mitochondrial medicine for aging and neurodegenerative diseases. Neuromolecular Med. 2008; 10:291–315.
- Reddy PH. Mitochondrial dysfunction in aging and Alzheimer’s disease: strategies to protect neurons. Antioxidants & Redox Signaling. 2007; 9:1647–1658.
- Savitha S, Sivarajan K, Haripriya D, Kokilavani V, Panneerselvam C. Efficacy of levo carnitine and alpha lipoic acid in ameliorating the decline in mitochondrial enzymes during aging. Clin Nutr 2005;24:794–800.
- Agadjanyan M, Vasilevko V, et. al.: Nutritional Supplement (NTFactor®) restores mitochondrial function and reduces moderately severe fatigue in aged subjects. JCFS 11(3): 23-26, 2003.
Share:
Related Posts
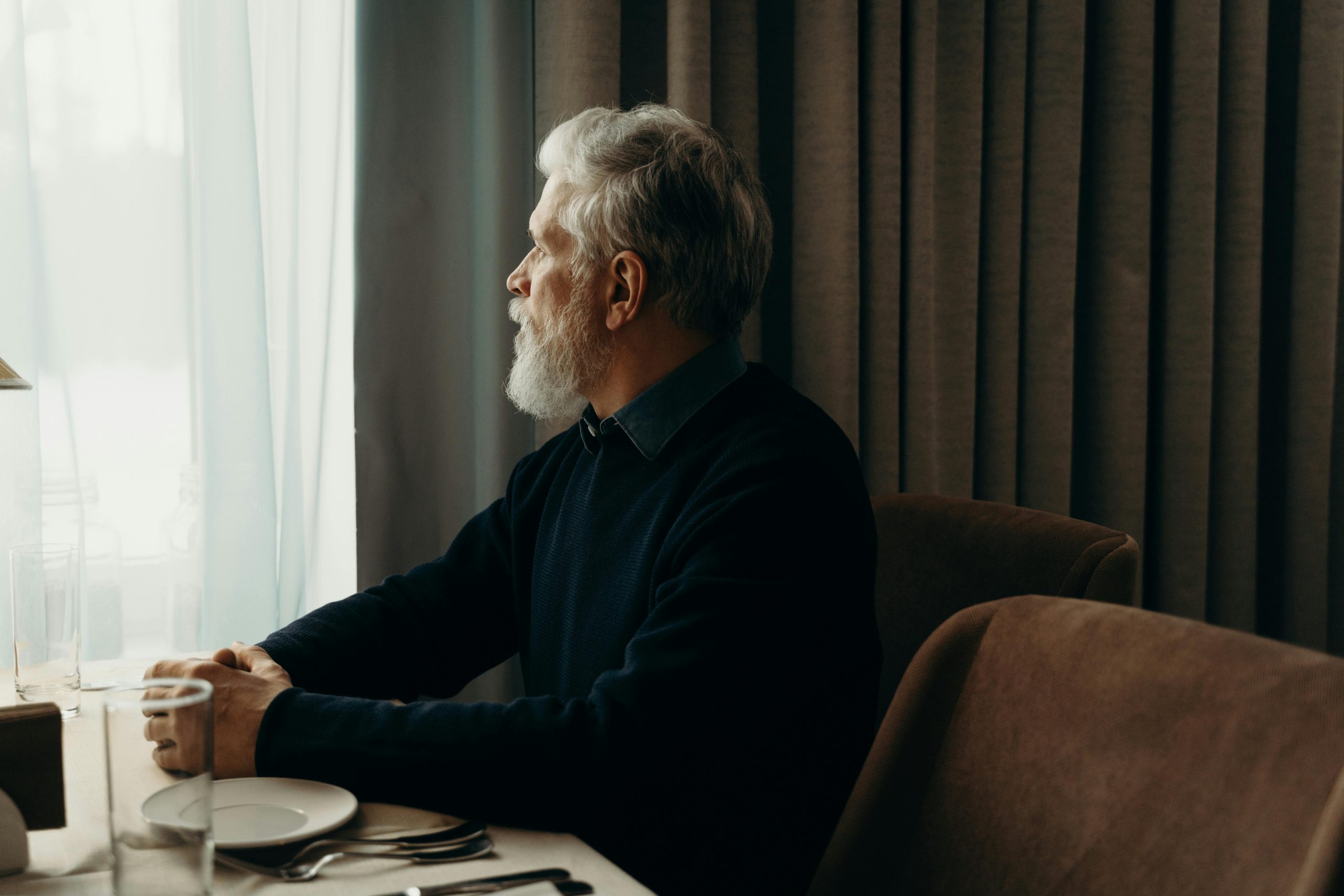
Why Would I Test Positive To Foods On A Dietary Antigen Test That I Don’t Eat?
Written by Precision Point Diagnostics | 2025 True food allergies are when the body develops antibodies against a particular food, or a related food. Patients
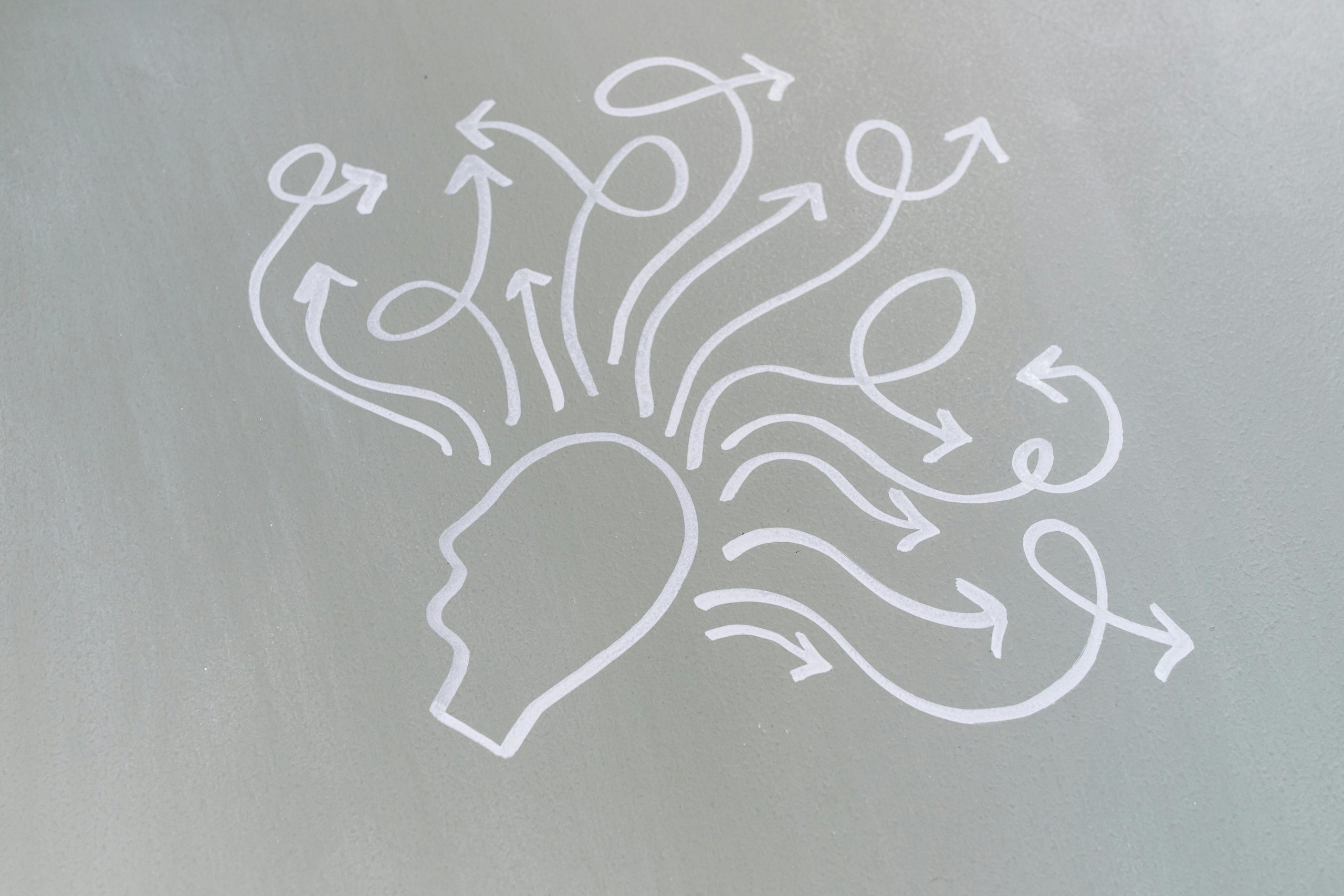
Mitochondria: The Overlooked Link in the Gut-Brain Axis
Written by Dr Sarah Daglis | 2025 The gut-brain axis is well-established, but there’s more to this dynamic relationship than meets the eye. A vital
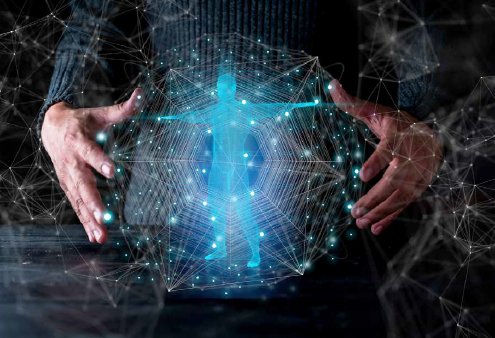
Unlocking Respiratory Resilience Through Functional Medicine
Article written by Dr Michelle Clark Naturopathic Doctor Clinical Education Manager, FxMed Published 1 September 2024 People take supplements to improve or optimize their health.
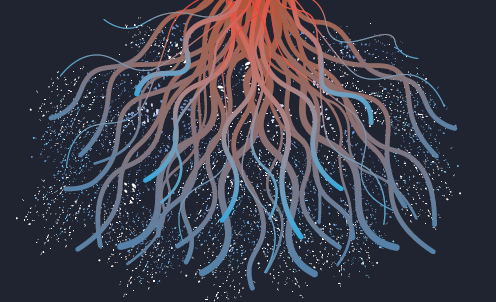
AHCC: Unlocking the Immune Intelligence of Shiitake Mycelia
Article written by Jessica Sanders BSc. (Psy), Dip Nat, Dip Med Herb Technical Projects, FxMed Published 1 September 2024 People take supplements to improve or
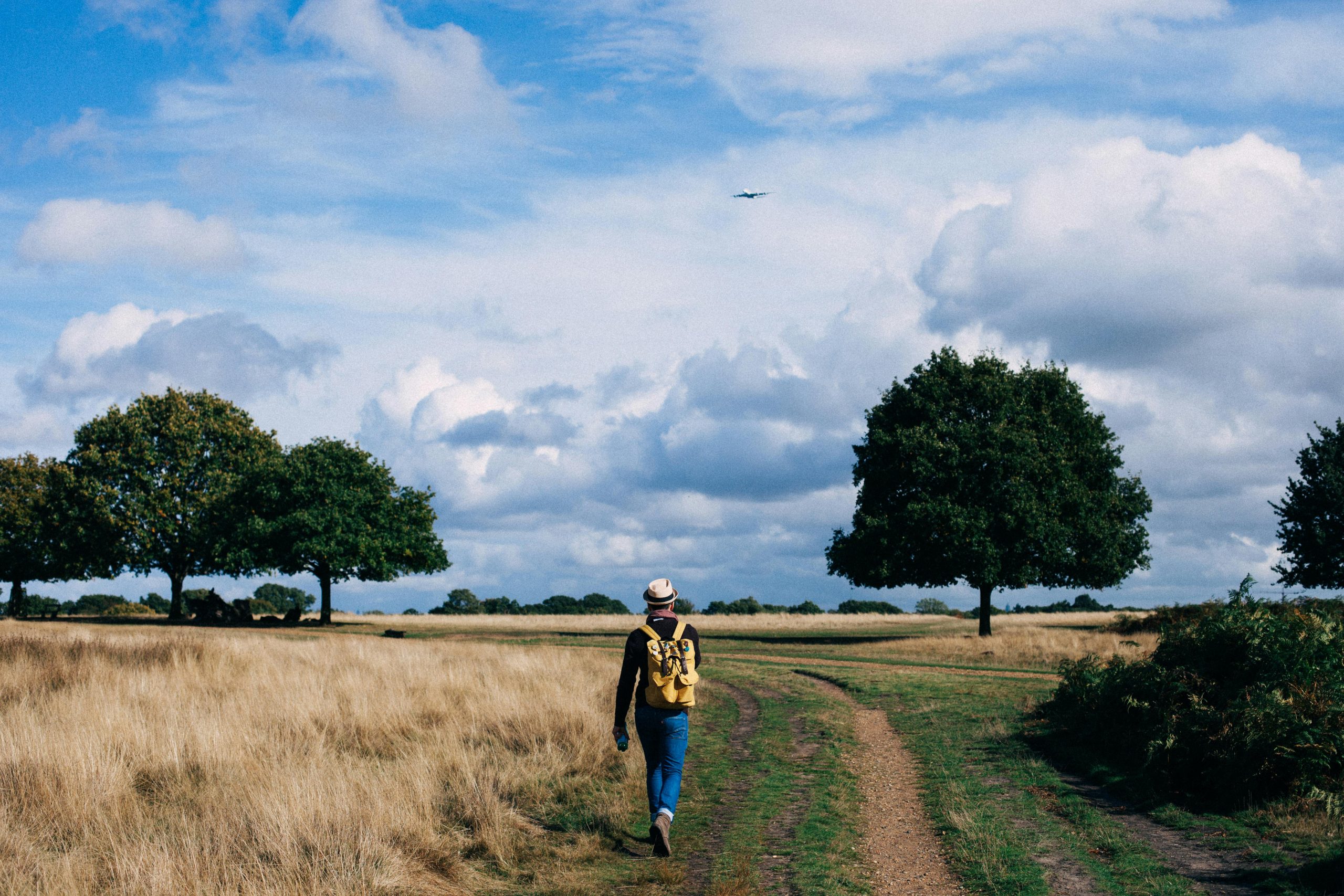
Life After Weight Loss Meds
Kathi Head, ND February 20, 2025 You would have to have been spending glorious hours lying on the beach on a desert island not to